Researchers use3D printingTechnology and nanotechnology have created a durable and flexible sensor for wearable devices that can be used for everything from monitoring vital signs to tracking sports performance. This new technology, developed by engineers from the University of Waterloo, combines silicone rubber with ultra-thin graphene layers to form a new material, which is an ideal material for making wristbands or running shoe insoles.
When the rubber material bends or moves, the highly conductive nano-scale graphene generates electrical signals, and this graphene is embedded in its designed honeycomb structure. “Silicone resin provides us with the flexibility and durability required for biomonitoring applications, and the added embedded graphene makes it an effective sensor.” Research in the Multiscale Additive Manufacturing (MSAM) Laboratory at the University of Waterloo Director Ehsan Toyserkani said, “It’s all concentrated in a single part.”
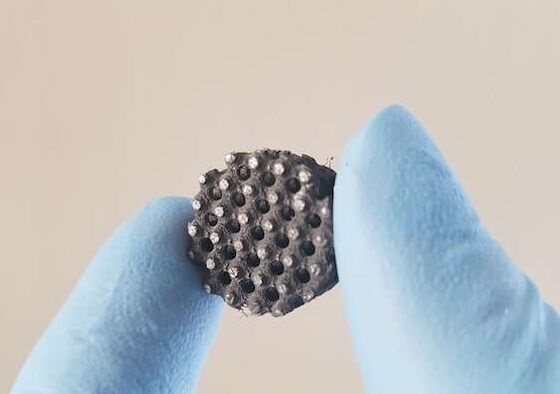
Only use the most advanced3D printingTechnology-also known as additive manufacturing-equipment and processes can produce a silicone rubber structure with such complex internal characteristics.
In addition to high conductivity, this rubber-graphene material also has extremely high flexibility and durability. “It can be used in the harshest environments, even in extreme temperatures and humidity.” said Elham Davoodi, a PhD student in engineering at the University of Waterloo and the project leader. “It might even be washed with your clothes.”
This material and3D printingThe craftsmanship enables the customized device to accurately fit the user’s body shape, while also improving comfort compared to existing wearable devices, and reducing manufacturing costs due to simplicity.
Toyserkani is a professor of mechanical and electromechanical engineering. He said that this rubber graphene sensor can be used with electronic components to make a wearable device to record heart rate and respiratory rate, record the force exerted by athletes while running, and allow doctors to remotely monitor patients. And many other potential applications.
Researchers from the University of California, Los Angeles and the University of British Columbia participated in this project.The latest series of papers in this research, “Making for Wearable Biomonitoring3D printingUltra-sturdy surface-doped porous silicon sensor” was published in “ACS Nano” magazine.
The development of wearable sensors
With the improvement of health care in the past few decades, residents of industrialized countries now live longer, but their health conditions are often complex and diverse. The survival rate of acute trauma has also improved, but this is related to the increase in the number of severely disabled patients. From an epidemiological point of view, the American “baby boomer” generation has now reached the age when it begins to exert severe pressure on the medical insurance system. Not only the United States, but also the healthcare systems of highly industrialized countries will face similar severe challenges.
These changing demographics raise some basic questions: How do we care for more and more people with complex diseases? How do we provide high-quality medical services to those who cannot access medical services? How can we maximize the independence and participation of more and more seniors?
Obviously, answering these questions will be complicated and will require us to change the way we organize and pay for healthcare. However, part of the solution may lie in how and to what extent we take advantage of the latest developments in information technology and related fields. At present, some technologies are promising to expand the capacity of the health care system, extend its scope to the community, improve diagnosis and monitoring, and maximize individual independence and participation. Wearable technology remote monitoring system is one kind, and the recent development of wearable sensor system has brought many exciting clinical applications.
Wearable sensors have diagnostic and monitoring applications. Their current functions include physiological and biochemical sensing, and motion sensing. Physiological monitoring can help diagnose and treat a large number of patients suffering from neurological, cardiovascular and pulmonary diseases (such as epilepsy, hypertension, dyslexia, and asthma). Family-based motion sensing may help prevent falls and help maximize individual independence and community participation.
Physiological monitoring of interest in rehabilitation includes heart rate, respiratory rate, blood pressure, blood oxygen saturation, and muscle activity. The parameters extracted from these monitorings can provide indicators of health status and have great diagnostic value. In the past, continuous monitoring of physiological parameters was only possible in a hospital environment. But today, with the development of wearable technology, accurate, continuous, and real-time monitoring of physiological signals becomes possible.
Integrating physiological monitoring in wearable systems usually requires clever design and novel sensor positioning. For example, Asada et al. proposed a ring sensor design for measuring blood oxygen saturation (SpO2) and heart rate. The ring sensor is completely independent. Wearing on the finger like a ring, it integrates technology to reduce motion artifacts to improve measurement accuracy. The applications of the ring sensor range from the diagnosis of hypertension to the treatment of congestive heart failure. Subsequently, the same research team developed an independent wearable sleeveless photoelectric pulse wave (PPG) blood pressure monitor. The sensor integrates a new height sensor based on two micro-electromechanical systems (MEMS) accelerometers to measure the hydrostatic pressure offset of the PPG sensor relative to the heart. The average arterial blood pressure comes from the PPG sensor output amplitude, which takes into account the height of the sensor relative to the heart.
Another example of ingenious design is the use of small wearable acoustic sensors (such as microphones) developed by Corbishley and others to measure respiratory rate. Place the microphone on the neck, record the acoustic signals related to breathing, and get the signal modulation package through band-pass filtering. Through the development of technology to filter environmental noise and other human interference, the researchers succeeded in achieving a respiration rate measurement accuracy of more than 90%. In addition, they also proposed an apnea detection algorithm based on the above-mentioned sensing technology.
In recent years, the development of the field of flexible circuits and the integration of sensing technology and wearable devices have benefited a lot from physiological monitoring. Patterson et al. introduced an ear-worn, flexible, low-power PPG sensor for heart rate monitoring. Due to the sensor’s location and unobtrusive design, it is suitable for long-term monitoring. Although this type of system has shown promising results, it seems that additional work is needed to achieve the reduction of motion artifacts. Appropriate reduction of motion artifacts is critical to the deployment of wearable sensors. Some problems due to movement can be minimized by integrating the sensor into the sleeve. Lanata et al. compared and analyzed different wearable respiratory function monitoring systems. The analysis results show that piezoelectric gas angiography has a better detection effect than spirometry. Nevertheless, further developments in signal processing technology to mitigate motion artifacts are needed.
Biochemical sensors have attracted great interest from researchers in the field of wearable technology in recent years. These types of sensors can be used to monitor biochemistry and the level of compounds in the atmosphere (for example, to facilitate monitoring of people working in hazardous environments). From a design point of view, biochemical sensors may be the most complex, as they usually require the collection, analysis, and processing of body fluids. Research progress in the field of wearable biochemical sensors has been slow, but with the development of micro-nano manufacturing technology, the pace of research has accelerated in recent years. For example, Dudde et al. developed a minimally invasive wearable closed-loop quasi-continuous drug infusion system that can measure blood sugar levels and automatically inject insulin. The glucose monitor consists of a new type of silicon sensor, which uses micro-perfusion technology to continuously measure glucose levels, and the continuous infusion of insulin is achieved through a modified advanced insulin pump. The device integrates Bluetooth communication functions for displaying and recording data, and receiving commands from personal digital assistant (PDA) devices.
With the support of the European Commission, a series of biochemical sensors have been developed as part of the BIOTEX project. Specifically, the BIOTEX project involves the integration of biochemical sensors into textiles for monitoring body fluids. In this project, the researchers developed a textile-based fluid collection system and sensor for testing the pH, sodium, and conductivity of human sweat in vivo and in vitro. Through in vivo and in vitro tests of the wearable system, researchers have proven that the system can be used for real-time analysis of sweat during sports activities.
As part of a similar project, ProeTEX, Curone and others have developed a wearable sensing suit for firefighters that integrates a carbon dioxide sensor to measure movement, environment and body temperature, position, blood oxygen saturation, heart rate and breathing rate. The ProeTEX system can warn firefighters of potentially hazardous environments and provide the control center with information about their health. The system developed by the above project can be used to design an electronic textile-based wearable system for remote health monitoring applications.
At the same time, people are becoming more and more interested in developing a “lab on a chip” system. Such a system can revolutionize point-of-care testing and diagnosis by making testing and diagnosis fast, inexpensive, and easily available. Wang et al. developed a system-on-a-chip (SOC) that integrates pH and temperature sensors for remote monitoring applications. Their SOC includes general sensor interface, ADC, microcontroller, data encoder and frequency shift keying radio frequency transmitter. Similarly, Ahn et al. developed a low-cost disposable plastic chip laboratory device for the biochemical detection of blood gas concentration and glucose and other parameters. The biochip contains an integrated biosensor array for detecting multiple parameters, and uses a passive microfluidic system instead of an active microfluidic pump.
(Editor in charge: admin)
0 Comments for “3D printing + nanotechnology to create flexible and durable rubber graphene sensors for wearable devices”