Scientists at Lawrence Livermore National Laboratory (LLNL) have developed a new method
biology
In a controlled mode3D printing, Expanding the potential of using engineered bacteria to recover rare earth metals, clean wastewater, and detect uranium.
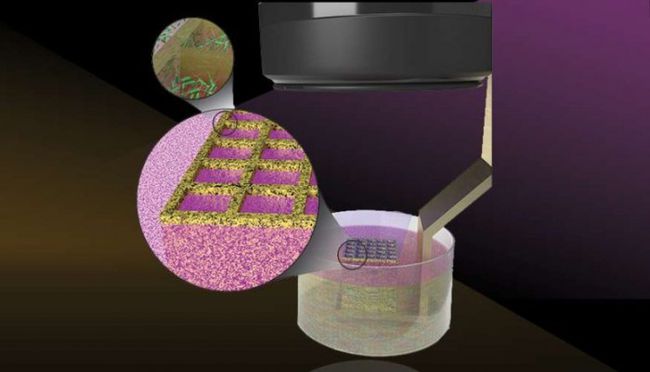
Through a new technology that uses light and bacteria-infused resin to produce 3D patterned microbes, the research team successfully printed an artificial biofilm similar to the thin layer of microbial communities that are ubiquitous in the real world. The research team suspended bacteria in photosensitive bio-resin, and used the stereolithography equipment (SLAM) developed by LLNL for microbial bioprinting3D printingThe machine’s LED light traps microorganisms in the 3D structure. This projection stereolithography machine can print at a high resolution of 18 microns, which is almost as thin as the diameter of a human cell.
In this paper published online in Nano Letters, the researchers proved that the technology can be effectively used to design a well-defined microbial population.They proved this3D printingThe applicability of the biofilm in uranium biosensing and rare earth biomining applications, and shows how geometry affects the performance of printed materials.
“We are trying to promote the development of 3D microbial culture technology,” said William “Rick” Hynes, the main researcher and LLNL bioengineer. “We think this is a very under-researched area, and its importance has not been fully understood. We Efforts are being made to develop tools and technologies that can be used by researchers to better study the behavior of microorganisms under geometrically complex but highly controlled conditions. By accessing and strengthening applications that have greater control over the three-dimensional structure of microbial populations Method, we will be able to directly influence how they interact and improve the performance of the system in the biomanufacturing process.”
Although it seems simple, Hynes explained that the behavior of microorganisms is actually very complex, driven by the temporal and spatial characteristics of their environment, including the geometric organization of the members of the microbial population. Hynes says that the way microbes are organized can affect a range of behaviors, such as how and when they grow, what they eat, how they cooperate, how they defend against competitors and what molecules they produce.
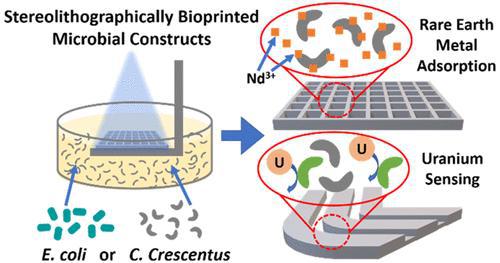
Hynes explained that previous methods of producing biofilms in the laboratory provided scientists with a small amount of control over the microbial tissue within the membrane, limiting the ability to fully understand the complex interactions of bacterial communities in nature.3D bioprintingThe ability of microbes will enable LLNL scientists to better observe the functions of bacteria in their natural habitats and study microbial electrosynthesis and other technologies, in which “electricity-eating” bacteria convert surplus electricity to produce biofuels and bio-fuels during off-peak hours. Chemicals.
Hynes added that at present, microbial electrosynthesis is limited because the docking between electrodes (usually wires or 2D surfaces) and bacteria is inefficient.pass through3D printingWith the combination of microorganisms and conductive materials in the equipment, engineers should realize highly conductive biomaterials and greatly expand and enhance the electrode-microbe interface to form a more efficient electrosynthesis system.
Industry is increasingly interested in biofilms. They are used to repair hydrocarbons, recover key metals, remove barnacles from ships, and serve as biosensors for various natural and man-made chemicals. On the basis of LLNL’s synthetic biology capabilities, LLNL’s researchers discussed the impact of bioprinting geometry on microbial function in the latest paper, where the bacterium Crescentium was used to extract rare earth metals through genetic modification. And detecting uranium ore.
In a set of experiments, the researchers compared the recovery of rare earth metals in different bio-printed patterns and showed that cells printed in a three-dimensional grid can absorb metal ions faster than in traditional bulk hydrogels. The team also printed a living uranium sensor and observed an increase in the fluorescence of the engineered bacteria compared to the control printing.
“The development of these effective biomaterials with enhanced microbial functions and mass transfer properties is of great significance for many biological applications,” said co-author and LLNL microbiologist Jiao Yongqin. “The new bioprinting platform not only improves system performance and scalability by optimizing geometry, but also maintains cell viability and achieves long-term storage.”
Researchers at LLNL are continuing their efforts to develop more complex 3D lattices and create new bio-resins with better printing and biological properties. They are evaluating conductive materials, such as carbon nanotubes and hydrogels, to transport electrons and feed bioprinted electrotrophic bacteria to improve production efficiency in microbial electrosynthesis applications. The team is also determining how to best optimize the geometry of the bio-printed electrodes to maximize the mass transport of nutrients and products through the system.
LLNL bioengineer and co-author Monica Moya said: “We are just beginning to understand how structure governs microbial behavior. This technology is a step in this direction. Manipulating microbes and their physiochemical environment to achieve more complex functions, There are a range of applications, including biomanufacturing, remediation, biosensing/detection, and even the development of engineered living materials-these materials are autonomous models that can repair themselves or perceive/respond to their environment.”
(Editor in charge: admin)
0 Comments for “Scientists develop a new method of 3D printing live microorganisms to enhance biological materials”